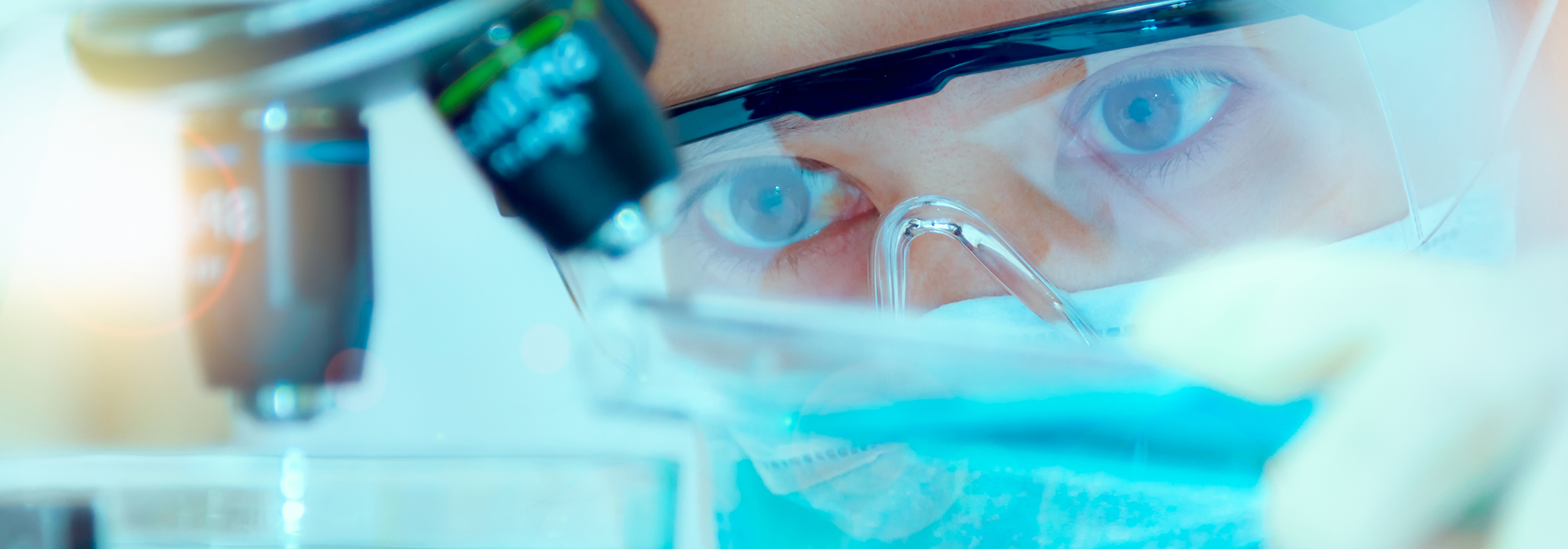
Generating, sharing and using digital data is key to accelerating new scientific discovery, innovation and efficiency in the global food system. Done well, digital technology adoption should enable Canada’s agriculture and agri-food sectors to support some of the most pressing Sustainable Development Goals adopted by the United Nations, including reducing hunger, mitigating and adapting to climate change, optimizing the use of arable land and water, and providing decent work and sustainable economic growth. It can also support food security and affordability in Canada.
Canada has a comparative advantage in food security and is a major supplier of food, feed and fibre to the world. Canada also has an advanced telecom sector and is an early innovator and adopter of many of the approaches farmers, scientists, industrialists, governments and NGOs are hoping will realize this potential.
Artificial intelligence, the Internet of Things, big data and gene editing offer the potential to increase production; reduce costs, emissions, water use, food waste and risk; improve resilience and food safety; and provide consumers with more information about the food they eat. New technologies and services are emerging in a wide range of areas relevant to agriculture, including precision agriculture, variable-rate technology, digital-farm management and digital animal-health management. There are significant opportunities for economic growth for technology developers, telecommunication companies, farmers and other companies along the supply chain from farm to table.
There are, however, many challenges and barriers to the development and adoption of these digital opportunities, including the readiness of the agriculture and agri-food sector to adopt new technologies, incomplete markets for data, industrial concentration and poorly defined data governance.
Canadian governments face a choice. They can follow the quintessential Canadian model of passive technology adoption, which is both low risk and low return. They can pursue a more proactive muddled approach, addressing easier barriers while ignoring the harder ones. Or they can go for broke, with an ambitious high-risk, high-reward strategy aimed at large-scale transformation.
The scale of the economic opportunity in growing global markets, combined with the societal benefits of greater global food security and a more efficient, lower-emission food-production system, justifies the ambitious strategy. This will require bold action on several fronts, in collaboration with the private sector and academic institutions:
Canada has the chance to succeed with a well-thought-out plan and the right mix of investment, planning, collaboration and governance. Canada can do well by doing good, increasing our economic returns from our world-class agri-food system, and contributing to global food security and climate change goals. There is no time to waste.
Canada is a major exporter of food and has an important role to play in achieving global food security. At the same time, the agriculture sector accounts for about 10 per cent of Canada’s greenhouse-gas emissions and is a major contributor to soil and water degradation. New technologies and services are emerging that offer the potential to increase food production, reduce costs and carbon emissions, improve food safety and achieve other benefits. But several hurdles stand in the way of their adoption. This paper assesses the challenges and barriers to the development and adoption of digital opportunities in agriculture and calls for an ambitious approach, led by Canadian governments, to optimize the use of these promising technologies.
Canada is a key supplier of food to the world, one of the top five surplus-producing and exporting countries and a leading-edge innovator. Canola crop yields have, on average, doubled over the last 50 years, while cattle and hog carcass weights increased by about 40 per cent between 1980 and 2015 with the development of larger breeds that finish at higher weights. By almost any measure, Canada has a legitimate and long-term role to play in achieving global food security. But as much as Canada is important for food security, it also has been a major contributor to the degradation of soil and water and a historical heavy emitter of greenhouse gases (GHGs). Canadian farmers contribute about 10 per cent of Canada’s GHGs, approximately half in the production of animals (mostly methane from fermentation and manure management, especially for beef cattle) and half from cultivating plants (especially nitrous oxide from fertilizer use) (see figure 1).
The good news is that many Canadian farmers are at the leading edge of developing and using new production methods that both increase productivity and contribute to Canada’s commitment to achieving net-zero emissions by 2050, as enshrined in the Canadian Net-Zero Emissions Accountability Act (S.C. 2021, c. 22). Dryland crops producers in the Prairies have adopted a bundle of agronomic, genetic and chemical innovations to improve productivity, and now are on average sequestering more carbon than they are emitting (Awada et al., 2021). Meanwhile, over the past 30 years, genetics and breeding improvements, combined with tighter management of the product life cycle, have resulted in production efficiencies for beef that have yielded a 14 per cent decline in CO2 per kilogram of live weight (Terry et al., 2020).
Digitization in the agriculture, food production and processing sectors is expected to enable technical optimization and help address societal concerns about farming, including greater product differentiation (Dawkins et al., 2017), new ways to manage animal health and welfare in livestock industries (Yeates, 2017) and data to control the environmental impact of different farming practices (Balafoutis et al., 2017; Busse et al., 2015). Getting there will require a mix of investments and policy innovations to overcome infrastructure gaps and market uncertainties.
It is not yet clear which technologies, if any, will prevail and provide the best outcomes for agriculture in Canada and around the world. There is so much diversity in the sector, in Canada and globally, that few producers face the same opportunities and constraints. This paper assesses the challenges and barriers to the development and adoption of digital opportunities in agriculture and explores what Canadian governments can do to optimize the use of these promising technologies.
Canada’s food system is inextricably linked to global production and trade. The global system is made up of hubs of production and centres of consumption that increasingly do not align. In many ways, Canada is not a centre for either, except in some discrete market segments. Nevertheless, Canada has a historical and growing comparative advantage in the export-directed parts of the farm and food system (especially grains, oilseeds and red meats), and a potential for higher-value production and food processing. The global system is stressed by rising and changing demands, and by ecosystem and climatic pressures, including soil and water resources. Canada is susceptible to many of these risks. One major complication is that agriculture is generally a low-margin industry with mostly limited market power. Innovation is both pervasive and vital, but the gains to research are modest and often widely distributed among innovators so that the incentives for rapid change are muted.
Agriculture in Canada varies widely, with approximately 190,000 farms in 2021 cultivating about 62 million hectares, equal to 6.3 per cent of Canada’s land area. Primary agricultural operators generate almost $32 billion of gross domestic product annually and employ about 241,000 workers. The primary sector exports abroad and provides feedstock to the domestic food and beverage processing and hospitality sectors, which collectively generate another $57 billion of GDP and employ more than one million workers (Agriculture and Agri-Food Canada, 2022a).
Agri-food activity is distributed quite unevenly. The largest 10 per cent of farms generate about two-thirds of all revenues. The nature of the output further defines the scale of farming ventures (Agriculture and Agri-Food Canada, 2022a). The 65,000 farms cultivating field crops realized $32.7 billion in farm cash receipts in 2021, or about $500,000 per farm, while 77,000 farms raising animals generated almost $30 billion ($390,000 per farm) and 17,000 horticultural enterprises posted $7.5 billion ($440,000 per farm) (Agriculture and Agri-Food Canada, 2022b).
Importantly, land and productive activity is regionally specialized. Saskatchewan alone has 47 per cent of all cultivated land in Canada, while Alberta and Manitoba cultivate almost another 40 per cent. Ontario has about 9 per cent of cultivated acreage, Quebec has 3.5 per cent, and the other five provinces and three territories combined have only 1.2 per cent of the cultivated land. The Prairies generate the lion’s share of cattle and calves, canola, wheat and pulses, which are mostly exported, while the other provinces differentially serve the domestic market — Ontario with dairy, horticulture and soybeans; Quebec with dairy, hogs and poultry; and the Atlantic provinces with potatoes and dairy (Agriculture and Agri-Food Canada, 2022b).
In the not-so-distant past, Canadian farms were small-scale, family-run operations that used few purchased inputs and mostly sold to local markets, co-operatives or public marketing agencies, which would then assemble and reposition volumes for local use and export. In many ways, these enterprises exemplified what some have now rebranded as “regenerative” or “circular agriculture” because they used animal power and farmer-saved seeds, all the while returning animal and green manure to the soil. While ecologically sustainable in their day, this model would not be able to meet current or future food needs.
A challenge for farmers everywhere is matching production with demand. In earlier periods, the supply chain managed this by blending and pooling, assembling appropriate quantities for the market through a mix of farmer-owned co-operatives, publicly managed marketing agencies and, in a few sectors, privately owned wholesalers. The supply chain attempted to deal with uncertainties and the opportunistic behaviour of actors through a range of intercompany investments and contracts. In Canada, the wholesale, food processing and retail system is integrated, with only a few enterprises bridging market demand and supply. For the most part, farmers were left out of these relationships.
But this type of agriculture is fading fast. Mechanization and the chemical revolution (fertilizers and pesticides) and genetics (hybrids, artificial insemination and genetic developments) have transformed how farming operates, delivering strong positive gains to yields and farm incomes, but at the expense of negative pressures on the local and global environment. At the same time, the domestic system of grain-marketing co-ops, food processers and government marketing agencies are engaged much more directly with farmers through a range of production and marketing contracts. This transformation is farther along in the U.S. than Canada, perhaps a signal of what is to come (Burns & MacDonald, 2018).
In the United States, about 90 per cent of chickens, 74 per cent of hogs, one-fifth of cattle, and 10 per cent to 60 per cent of various plant crops are raised under production contracts (Whitt, 2022). Farmers under contract typically use their own land, labour and capital but are provided with specifically selected genetics and breeding materials (semen, hatchlings), feed and medications, production plans (feeding ratios and target weights), and instructions on when and how to deliver their product. Some contracts also direct farmers on how to deal with health risks, manure and other waste.
Farmers producing crops, fruit and vegetables are more likely to enter marketing contracts, which usually set a price (or a pricing formula), product quantities and qualities, and a delivery schedule. Contractors increasingly offer bundles of inputs and financing, but farmers usually have full discretion to decide how to meet the contract. Marketing contracts are much less common than production contracts: about 45 per cent of U.S. fruit and 40 per cent of vegetables are contracted, but dryland crops such as maize/corn and wheat have less than 15 per cent of the area under marketing contract (Whitt, 2022).
Two pressures are driving tighter relationships in the supply chain. First, regulators require greater management of quality, risk and liabilities. Food safety failures in key markets have driven regulators, retailers and large food processing companies to invest heavily in hazard analysis critical control point (HACCP) programs, segregation and traceability systems to ensure the safety of their products (Smyth & Phillips, 2003). In the early stages, the goal was simply to avoid doing harm but, increasingly, firms have found that these systems can drive efficiencies and deliver more differentiated foods to serve specific consumer segments. Food wholesalers and retailers now promise and deliver foods that comply with a range of organic, halal, kosher, ethical and environmental standards, all which offer the possibility of extracting a premium from the discriminating consumer.
Data also increases productivity, improves operating margins, manages risks and tightens relationships in the supply chain. At the most basic level, greater use of information from digital sensors and platforms offers prospects for continual improvement of agricultural processes. The digital system offers established farmers access to important information including seed and animal characteristics, climatic and weather patterns, soil conditions, past agronomic performance, inventories and market opportunities. This allows them to improve farm performance and more effectively integrate their production with the supply chain.
Beyond improving productivity, digital systems promise to help gauge and address risks arising from environmental stresses and market variability. If this works, farmers should be able to proactively respond to conditions to protect short-term revenue and, over the long term, work to enhance soil and environmental quality management at the farm and system level (Shen et al., 2010).
As in much of the economy today, there is significant hype about what “cyber things“ could do for the agricultural sector. Undoubtedly, there are opportunities, but where and how digital applications fit in the agricultural system requires careful consideration. Digital platforms (Gawer & Cusumano, 2014; Kenney & Zysman, 2016) in agriculture require a different conceptualization than social media platforms like Facebook and X (formerly known as Twitter), or app-based companies like Uber and TaskRabbit (Friedman, 2014). Unlike industries and clusters based in manufacturing and services, which can be fragmented and modularized across global value chains (Wixted, 2009), agriculture involves assets that are immobile — especially cultivated land and related improvements. Over the long-term, the threat to the sector will come not from foreign competitors but from local and national competition for land, labour and capital from other sectors that are growing and becoming more productive. Nevertheless, agriculture will look and feel different as digital applications make inroads, and new platforms, systems and landscapes emerge.
Conceptually, the biggest returns are projected to come from data that is derived from and combined with sensors, processes, algorithms and institutions that allow farms and the food system to do things better or differently (Pavitt, 1998; Wolfert et al., 2017; Zuboff, 1985). The scale and scope of change are characterized by the so-called “six Vs” of the big-data world: volume, variety, velocity, value, veracity and visualization. The challenge for agriculture is that currently about 95 per cent of data generated is in an unstructured, raw format, and few if any firms or consumers are deriving much sustained net value from the application of data in the production and marketing system.
Most of the things digital applications have been applied to are already reasonably well organized, so the incremental benefits have been modest relative to the capital costs of the new applications. Early adopters must have adequate resources and scale to make adjustments so that the technology delivers value, which will differentially privilege larger and more established operators. As with most early-stage innovations, net gains should grow over time as adoption rises and the upfront costs are amortized over a larger market.
The vision is that the digital revolution will bring about a cohesive, effective, sustainable and responsive system. Studies suggest that the use of digital data, cloud-computing technology, and big-data analysis in the realm of digital or smart farming enhances productivity and income. These tools can enable farmers to make more precise decisions through machine-generated instructions and utilize real-time, site-specific information to streamline field operations and itineraries (Internet of Food & Farm, 2020; Poppe et al., 2015; Sundmaeker et al., 2016).
One area of focus is on breeding, feed and seed. According to one view, the combination of real-time data moderated by artificial intelligence will advance breeding in ways that deliver infinitely differentiated, timely new varieties and pedigrees that will mitigate the limits on productivity and deliver novelty in our foods.
This is still a work in progress. There are various teams in Canada and abroad working on this (for example, the Plant Phenotyping and Imaging Research Centre in Saskatoon). Despite promising applications, challenges remain in utilizing the potential of real-time data for crop improvement and breeding. The adoption of digital tools in breeding is still in its infancy and integrating data into breeding programs creates problems not yet clear to many breeders. The need for big-data management tools is crucial (Awada et al., 2018).
One early prospect is the combination of data-enhanced breeding strategies with gene editing (such as CRISPR-Cas9). According to one estimate, this could reduce the cost and time so much that the break-even acreage is reduced by 96 per cent compared with genetically modified crops (Bullock et al., 2021). This would create a host of niche applications that could whittle away at the commodity production space, replacing it with high(er) margin possibilities.
A second area of focus is on sensors, GPS-managed equipment and artificial-intelligence (AI) instrumentation, which will enable farmers to manage their farms while reducing their environmental footprint. Commercially cultivated plants currently realize only about one-quarter of their theoretical biological yield because the genetics are not optimally matched with microclimatic conditions and farm operations. Precision agriculture — doing “the right thing, in the right place, in the right time and in the right way,” using a suite of tools (Pierpaoli et al., 2013, p. 62) — emerged in the 1990s and is now embedded in the current system (Bramley, 2009; Hall, 2017). Precision agriculture involves the monitoring, control and treatment of animals and crops at the unit level to manage the spatial and temporal variability of soil, crop and animal factors (Sundmaeker et al., 2016).
In the crop sector, digital services will facilitate the systematic customization of processes to manage production operations, such as seed-mix combinations and proportions, timing, sources and placement for seeds, fertilizers (and other chemicals), along with tillage and harvesting practices. This targeted approach reduces risk and enhances production efficiency (van Es & Woodard, 2017; Wolfert et al., 2017). Done properly, it would transform every field into a crop trial, providing additional research information. Beyond cost savings and productivity gains, the efficiency brought about by smart farming can also reduce waste and alleviate land degradation and greenhouse-gas emissions resulting from unsustainable practices and inefficiencies (Soto Embodas et al., 2019).
At the other end of the food chain, there are opportunities for consumers to become involved, by providing insight into what they value and want to buy. Supply-chain logistical integrators can combine consumer and farm data to deliver just-in-time products to processors and consumers, reducing the 30 per cent of food wasted globally in the supply chain and in household fridges.
This has already started to happen. AgFunder, an online venture capital investment platform, attracted US$150 billion from 2018 to 2022. Investments in climate technology directed to farms accounted for about 35 per cent of all investment, rising to over half of the activity in 2022. Investments in consumer food technologies (such as e-grocery and in-store technologies) attracted about 56 per cent of this investment over the period, albeit only about 40 per cent in the latest year. Investments in food safety, traceability, logistics, transport and processing represent only about 10 per cent of the activity in the past half-decade (AgFunder, 2023). U.S. companies make about 42 per cent of all the investments while Canadian firms account for only 4.4 per cent. The sector appears to be maturing and there has been a shift to late-stage investments from “seed stage” deals.
The scale of the data challenge is growing rapidly. Vast amounts of data have been collected in recent years. In addition to a large volume of historical weather, market and farm-level production data, the breeding system is transforming into a data-rich system. The University of Saskatchewan’s Plant Phenotyping and Imaging Research Centre, for example, has been generating terabytes of data annually, including a mix of microclimate measures, soil tests, phenotypic measures from field trials, crop imaging and genetics. The centre reports accumulating around 230 terabytes of data since 2016, with 100 per cent to 120 per cent growth every year. About 90 per cent of the data in its PlotVision platform is image data, of which about 70 per cent is processed drone images. The other 10 per cent consists of information from the fields, GPS data for flights and analysis metrics for researchers. It provides information for various crops and the aggregators link up data from sensors in farm machinery, in the supply chain and in the global ecosystem.
While digitalization is underway, it is far from clear if it will offer enduring value. This means the policy response is crucial for defining the future.
Realizing these visions will take investment and policy innovation. Four particular policy challenges could limit the uptake and use of digital applications in agriculture: industry readiness to adopt new technologies; incomplete markets for data; industrial concentration and uneven distribution of gains; and poorly defined data ownership and governance.
A mixed set of attitudes and capacities influences the use of technology. The 2021 agriculture census revealed that producers are on the way to actively adopting technologies that provide precision in farm management. About half of grains and oilseeds farmers were using soil testing and auto-steer, with about one in four using variable-rate input application and geographic information system mapping. Fully robotic milking was used by about one-quarter of dairy producers, while only about 7 per cent of greenhouse operators used robotic equipment (see figure 2). Although the information is not available, one might expect that most of the early adopters are the larger and better capitalized operations.
However, the adoption process is far from linear. Rogers (2003, p.172) describes the process as “an information-seeking and information-processing activity, where an individual is motivated to reduce uncertainty about the advantages and disadvantages of an innovation.”
Rogers describes the adoption process as having five stages: awareness, persuasion, decision, implementation and confirmation. Ugochukwu and Phillips (2018) identify a host of other critical factors including the compatibility of the new technology and evaluations of the benefits and costs of adoption. These can be affected by the subjectivity of the risks, rewards, timing and social acceptability of the technology.
Both the ability to trial a technology and the subjective evaluation of the benefits, costs and risks are hurdles to adoption. Smaller enterprises are often unable or unwilling to invest their time in testing the latest technology. As a result, there is a distribution of adopters resembling a bell curve.
Innovators and early adopters tinker with the new technology and make it work in new settings. They are followed by the majority of users, representing about two-thirds of the market. Eventually, the so-called “laggards” embrace the new technology.
Theory and evidence suggest that innovators and early adopters are more likely to be better educated, and have higher incomes and stronger social networks. In Canada, many of these will be the 4 per cent of farmers who generate more than half of total sectoral revenue (Chen & Clark, 2023). In agriculture, digital technology takes a lot of investment both in learning by doing and in investing in assets (like sensors and algorithms), and these won’t have value if they fail to deliver or don’t offer economies of scale. This means that larger operations are more likely to be able to risk adoption.
A survey of innovation and business strategy reveals that agriculture industries and downstream handling and processing sectors were more intensively innovating between 2017 and 2019 than primary producers and at a rate above the national average of all firms (Statistics Canada, 2021). However, given the data in figure 2, the gap may be closing. The survey also examined the obstacles to innovation. Farmers reported fewer economic concerns and instead, singled out uncertainty and risk, lack of skills and market size as significant barriers to adoption.
Other related concerns included low-probability but high-cost risks. For example, the ability to better program and differentiate performance might destabilize the regulatory and insurance systems, which tend to focus on average farm performance rather than individual farm performance. As differences in individual farm performance increase, the approach could be problematic. Some farmers fear they may face tighter underwriting conditions while the entire agriculture sector is concerned about compliance with market rules because regulators have a greater capacity to identify, trace and assign errors and failures to individual actions (Wiseman et al., 2019). This need not be a roadblock to adoption, but a better signal of if and how farm-level data will be used in program design, implementation and enforcement can and probably should be part of any data policy.
As well, there are concerns about the security of data itself. The rise of cyber risk, including denial-of-service attacks, phishing, ransomware, Trojan horses, viruses and malware remain an ongoing issue. As more digital platforms are wirelessly linked through cloud computing, there is more exposure to threats. None of these are reasons to avoid the technology, but they are considerations that are being watched carefully. Including data security plans at both the farm and system level will be necessary, and over time should be integrated in federal-provincial-territorial plans, policies and programs targeted to farm resiliency.
While Canada has an advanced telecom sector that is well capitalized and innovative, there are some gaps in the service. One major challenge is poor rural connectivity. Canada’s Auditor General (2023) reports that in 2021 almost everyone in urban settings had access to minimum internet speeds, but only about 60 per cent of rural and remote areas had access to internet speeds needed for running the sensing and monitoring systems that drive digital agriculture. Governments and telcos are investing in improving this and Starlink, an internet service provider, now offers roaming/mobile internet service across a large part of rural Canada. The speed (and cost) at which rural connectivity happens will determine the rate of digital change in the agri-food system.
Rural connectivity is simply a matter of will and resources. Federal and provincial governments must work together to sort out the appropriate financing of a more extensive rollout. Currently, the focus seems to be on encouraging existing Canadian-based telcos to make investments in providing better service to rural and remote communities. Other remote, underserved regions in Africa and some communities in northern Canada have found that satellite delivery is a faster and more cost-effective approach. Telesat has developed a network of 198 Low Earth Orbit satellites, integrated with on-ground data networks to begin to offer services in Canada and abroad (The Peak, 2023). Starlink already has an active satellite network and marketing program that targets farmers engaged in precision farming in Australia and the U.S., and the Canadian Federation of Agriculture (2023) partnered with Starlink in spring 2023 to offer an introductory package to Canadian farmers. There are undoubtedly a range of options that can and should be explored to rapidly expand capacity at least in Canadian farming regions.
It is hard to generate a price for data. Valuing anything new, not just data, is fraught with challenges. Without a clear and transparent value proposition, markets tend to fail. Even if there is a valid price, adaptation is necessary to fit the data to individual needs. Not everyone benefits equally. There will inevitably be some early adopters who will blaze the path for others. Overall, the variations in respective valuations by suppliers and users will mean that markets could fail to emerge.
Large data sets power smart farming platforms. A data set’s accuracy and efficiency in predicting statistical outcomes and providing services increase when there are more variables. Economies of scale and scope emerge from large, fixed costs and low costs of collecting and processing additional data (OECD, 2021). Large international firms have competitive advantages in collecting and aggregating data across farms, crops, locations and inputs because of their investments and established networks.
The high fixed cost of data collection and aggregation creates barriers to entry for new firms. This means that a few firms dominate a specific data market, similar to other digital platforms like Google and Amazon (Koutroumpis et al., 2020; Shapiro & Varian 1998).
This also creates an imbalance in the data market. The value of individual farm data is low, and there are diminishing returns to scale and scope. Farmers often don’t receive much of a return for sharing their data in the market, but they still pay for data-driven services (Atik & Martens, 2020).
Economies of scope in reusing data can be achieved to produce new types of products or services. Multinational firms can spread fixed costs over a variety of products or services, which can work as a barrier to entry for small firms. The sharing, joint utilization and selling of data can also create economies of scope but could lead to economic losses for the original firm if they generate competing services or products.
Awada and Phillips (forthcoming) built a model that measures the distribution of surplus from data-driven technical change. The simulations demonstrate that farmers who provide data receive a very low surplus regardless of the market structure. Nevertheless, private rights are important for agriculture technology producers (ATP) themselves and for the general uptake and use of the technology.
With assigned rights to data, consumers and ATPs share the bulk of the returns. Without any private ownership of the data, the return to consumers increases slightly from 34 per cent to 35 per cent and for landowners from 20 per cent to 34 per cent, while the return to ATPs drops 96 per cent — but no ATP would invest under these conditions. What results is that good development is blocked by incomplete markets.
It can be challenging to strike a balance between equity and efficiency in the technology market. While reducing concentration among producers may help farmers, some level of concentration is needed to ensure the development of new technologies. The difference between the value of data perceived by farmers and its actual market value can be so great that it impairs the market’s ability to generate useful data. This gap can be attributed to the endowment effect, where farmers value the data they hold more highly than the data they have to buy (Jobe, 2019). To address this challenge, policymakers must find ways to promote more equitable and efficient markets.
Data markets are incomplete mostly due to a lack of clarity of prices. Prices for digital products are poorly managed in all parts of the sector. The recent disruptions in digital currencies illustrate the harms that can arise from non-transparent pricing systems. A mix of regulations and appropriately structured tax measures or subsidies should be considered to advance transparent data markets in Canada.
Right now, most farm data contracts are confidential. Understanding prices would help set conditions for new capital investment and more efficient contracting. In the past, the federal government encouraged the development of transparent pricing in commodity, stock and debt markets. Transparent commodity trading, with bid and offer prices and volumes and timely reporting of effected transactions, is core to creating an efficient and effective market.
Big-data algorithms, ontologies and norms offer significant, positive externalized network effects that can create value for innovators and users. The challenge is that network effects can and often do create lock-in, which can block successive innovation and increase the market power of the innovator. This has already happened with market leaders such as Alphabet, Amazon, Apple, Meta, Microsoft and their Chinese analogues, which control the lion’s share of their specific markets.
Canada’s lack of leaders in the general digital world and among the leading firms investing in agri-food innovation is a disadvantage. The U.S. dominates in almost all the key segments, while the EU and China are duelling for regional influence. While there is much to gain from connecting to global leaders, much can be learned from Brazil and Argentina, which have recently engaged more responsively to new technology and gained modest benefits.
Digital-data markets, including those related to agriculture, often present significant barriers to entry, resulting in market power for incumbent firms (Atik, 2021; Birner et al., 2021; OECD, 2020). These barriers can be exacerbated by mergers, acquisitions and partnerships among multinational corporations. The digital agriculture industry has seen several large-scale mergers, including Bayer and Monsanto’s US$63-billion merger in 2018, and Dow Chemical and DuPont’s US$130-billion and ChemChina and Syngenta’s US$43-billion mergers in 2017 (Verdonk, 2019).
Prior to the merger, Monsanto had already acquired several leading precision-planting equipment firms. It also invested in emerging agricultural technology businesses, including AquaTEK and AgSolver. Similarly, Bayer acquired ProPlant, a farm-management software, sensing and Internet of Things provider, and Zoner, a geo-information system from IntelMax.
In 2015, a subsidiary of Monsanto launched Climate FieldView, which now serves as the platform for Bayer’s digital activities. It provides agronomic services to farmers, which guide their decision-making process through digital software tools that interact with a farmer’s agricultural equipment. In 2021, the platform was used on more than 180 million acres (72 million hectares) in over 23 countries, and in 2022, Climate FieldView partnered with more than 34 industry partners, including sensors, imagery, dealer solutions, grain trading, farm-equipment manufacturers and insurance companies (Climate FieldView, 2022). Climate FieldView has also signed application programming interface agreements with equipment companies for data exchange for field- and site-specific documentation. Recently, the company partnered with Microsoft Azure to develop cloud-based digital tools and data-science solutions to support sustainability across the food supply chain (Bayer Global, 2022).
In addition, Bayer launched a decarbonization program for agriculture in the U.S. and Brazil in 2020 and in the EU in 2021. Through the Climate FieldView platform, Bayer plans to offer a digital tool that enables farmers to claim carbon credits based on verified field data and accurate estimates of stored soil carbon (Bayer Global, 2022).
These and other merging conglomerates have decreased the number of players in the market and have created behemoths, which concentrate data in a few often-incompatible systems. This creates lock-in, which is a major barrier to entry for small startups, co-operatives or software developers not aligned with the large players. With limited budgets to cover the high fixed costs of data collection, integration processes and analysis, new entrants find it difficult to compete with established conglomerates like Bayer, which can both skew the distribution of benefits and narrow the types of innovation that are developed and used. In effect, the established system generates a path-dependent world.
If these firms continue to dominate, there will be implications for policy. First, policies and mechanisms to facilitate and maintain access to the latest machinery for everyone should be considered. The U.S., China and the EU host the major manufacturers but some countries, like Canada, operate in niche areas that provide access to the system. Canada’s particular strength is in specialized farm machinery equipment that is towed, especially air seeders. This places the industry in a good position to advance variable seeding technologies. Countries without indigenous machinery industries will have a more difficult time incentivizing the development of technology that is attuned to local needs.
Second, because fear of losing autonomy is one of the major barriers to farmers fully adopting these technologies, federal, provincial and territorial governments should become more engaged in data regulation and privacy issues. Arguably, only the U.S. has dominant agricultural players, so most other countries may have an interest in working together to design policies that sustain access to aggregated data and their related returns.
Lastly, concentrated industrial structures, such as the Bayer system, increase the possibilities of highly disruptive cyberattacks in agriculture. This could conceivably elevate farm policy to the top of the national if not international security agenda in coming years.
As mentioned, Canada and other second-tier players in digital agriculture could look for lessons to countries that are not competing to be first movers. For example, Brazil and Argentina have invested heavily in responsive regulatory systems to accelerate the uptake and use of the latest genetic and production technologies in their key crops. Their logic is that, even if they are not targeted as innovators by technology developers, they can speed up market demand by quickly and efficiently reviewing and approving new technologies. Faster uptake increases revenue for technology developers and delivers higher margins for early adopters, which can encourage multinationals to target breeding and technologies to the specific needs of these smaller markets (Smyth, 2022). In effect, they take the “if you can’t beat them, join them” approach. Canada should adopt some of these tactics.
There are three ways governments can work to mitigate the effects of industrial concentration. First, Canada has a few homegrown ventures with a decent foothold in the market, which could offer some competition to the global aggregation that we are seeing.
Perhaps more importantly, Canada could exert more effort, both individually and in collaboration with like-minded countries such as Australia, to manage the contestability of the markets. Whether the market is served by a domestic or foreign firm, the only real check on market power is that it is contestable. The inherent lock-in of digital markets is at the heart of the issue. One way to address this is with regulations that improve data interoperability through specification standards for metadata, vocabularies and keywords. This will require a fair bit of work and investment.
Successive innovations are the best check on market power. Brazil and Argentina have decided to work with whichever firm has a product ready for use and have structured their regulatory systems to undertake their reviews as quickly as is safe. This ensures that, while they may not own the intellectual property, they gain higher returns as first movers and adopters. Over time, there is a sense that companies investing in innovations are considering the needs of these early adopters in their research plans. Canada has an excellent regulatory system for agriculture and food innovation, but it is too slow. Studies suggest that every year of delay can reduce returns on investments on research and design by up to 2 per cent, which is a major drag on investment (Smyth et al., 2014). The Health Canada Pest Management Regulatory Agency, the Canadian Food Inspection Agency and Health Canada should strive to deliver the fastest safe decisions in the world. While that will require new processes and more funding, it would encourage innovative firms anywhere around the world to see Canada as an early adopting market and a target for cutting-edge technology, which would offset the anticompetitive nature of digital transformation.
Data governance refers to “the collective set of decision-making processes for the use and value-maximization of an organization’s data assets” (Pierce et al., 2008, p.7). Scholars (e.g., Brous et al., 2016; Khatri & Brown, 2010; Otto, 2011; Weber et al., 2009) refer to data governance as the specification of accountability frameworks — policies, guidelines, standards and practices — and decision rights that shape people’s behaviours about the use of data. Data governance creates standards and guidelines for data quality management, defines roles and allocates responsibilities for decisions (Weber et al., 2009). Effective data-management and sharing practices require that data should be properly governed using appropriate institutional frameworks.
The question of data ownership is an entry point to exploring the governance challenge. Who owns and controls the underlying data that drives the algorithms and value of digital agriculture may be the most important policy variable (Bronson, 2018). Unambiguously, data ownership, management and control are at the heart of the digital play in agriculture, as in every digital transition. The industry is highly engaged with these questions, but unlike consumers or large enterprises that may simply look for the welfare-enhancing uses of data, farmers see data as a core asset of their operations.
Data ownership in agriculture is complex and evolving. While data itself cannot be patented, copyrighted or trademarked, the algorithms, visualizations and business models that use data can be protected by property laws. Data in smart farming can be classified as non-personal under current data laws, allowing for ownership and rights to be traded and transferred from farmers to agriculture-technology providers through contracts.
To address this issue, the American Farm Bureau Federation (2014, 2016) and other organizations developed a set of principles for agriculture technology providers to ensure that farmers own and control the information generated by their machines. Additionally, various agricultural data certification plans, such as those in Canada, New Zealand and the EU, attempt to address these issues but may have limited effectiveness without legal support (Sanderson et al., 2018).
Even if legally enforced, data-sharing codes of conduct do not guarantee the ownership and data rights for farmers. The superior bargaining power of agriculture technology producers in contractual negotiations can significantly influence the terms of the contract, ultimately determining who possesses effective ownership, access and usage rights over the data (Atik, 2021; Atik & Martens, 2020; OECD, 2020).
One example is agricultural machinery, which is equipped with sensors and interfaces that give exclusive rights to farm-level data to the machinery manufacturer. Dependence on that data locks farmers into one provider and weakens their negotiating power. Even when portability mandates are included in data contracts, some ATPs may make user switching difficult and costly by using incompatible formats and protocols. Exclusive control over historical farm data impedes entry of new firms that lack access to nonreplicable or substitutable data.
In addition to exclusive access, service providers collect and store historical farm-specific records for their clients, giving them extensive de facto control (Atik & Martens, 2020). In crop production, ATPs dispense services through apps on mobile or proprietary data-interface devices. Farmers are locked in once they buy a device, particularly if this device uses non-interoperable data formats and software design. These then provide market power to incumbent ATPs.
Legislation or regulation at either the national or provincial level will be challenging, as there is little likelihood that rules can drive competing systems to converge on a single format. When this has happened in other digital sectors, it has arisen from the strong network effects of dominant players, and not from the actions of regulators. The downside of relying on this approach is that it will further enhance the market power of the operator, which then becomes the industry standard.
Few operators can completely utilize the data they own by themselves. Sharing is necessary but faces institutional, legal and technical barriers. These obstacles arise from the lack of governance and stewardship (Awada et al., 2021). To ensure quality, accessibility and usability, it is essential to establish accountability and oversight mechanisms, as well as an operational framework for efficient collection, analysis, ownership and distribution. By doing so, semantic, technical and legal interoperability can be achieved, enhancing the potential for data exchange and reuse (Awada et al., 2021).
Even when developed by public scientists, most data remains in the custody of research institutions and is by default not open. Yet most researchers accept that open access and exchange of data offer the best prospects for advancing science. In response, a group of scholars developed and published the “FAIR guiding principles” to advance more structured and purposeful data management and stewardship in agriculture (Wilkinson et al., 2016). FAIR stands for “findability,” “accessibility,” “interoperability” and “reusability”; these principles are used to guide producers and publishers in maximizing value from the algorithms, tools and workflows that led to the data.
FAIR guiding principles have operated in some academic institutions in Europe for the better part of a decade, and since 2016 they have been endorsed by the G7 (2017) and a range of science funding organizations and national governments. They define criteria that data must meet before being deposited in a digital repository to enhance reproducibility and reusability.
These principles apply to all digital resources — data, software, protocols, images, repositories, web services and others arising from research — which must be associated with a unique and persistent identifier and rich metadata (for example, contextual and supporting information) to enhance discovery, accessibility and reuse (Dumontier & Wesley, 2018).
Each of the principles leads to a specific, actionable policy (Ugochukwu & Phillips, 2022). Making data easily found implies that potential (re)users can locate the data online. To find or discover data, a globally unique and persistent identifier — such as a digital object identifier (DOI), uniform resource locator (URL) or archival resource key (ARK) — must be attached, including rich metadata that specifies the unique and persistent identifier, catalogued or registered in a searchable resource (like Google) and published in a credible and rightful repository.
Data is often made accessible by both humans and machines via repositories. From a practical perspective, interoperability — the “ability of two or more systems or components to exchange information and to use the information that has been exchanged” — requires technical compatibility of format and protocols, which requires institutional (legal and regulatory) frameworks and metadata practices (IEEE, 1990). Any framework will need to specify standards for security and protection, what can be shared and integrated, and how information can be shared with other organizations within the ambit of the law — potentially through a memorandum of understanding.
The use of community-accepted and recognized specification standards (schemas) for metadata, vocabularies and keywords that precisely define concepts and qualities is key to enhancing data interoperability. A standard metadata schema describes elements (e.g., title, description, date of issue or modification, publisher, licence and keywords) that should accompany a data set within a domain of application. Data sets and metadata should be prepared using appropriate common, standardized and recognized open-file formats for easy integration. The establishment and adoption of standard controlled vocabularies and classifications at the design stage eliminates ambiguities and strengthens semantic interoperability and exchange of knowledge.
Data reuse is contingent on several factors, including its discoverability, accuracy, clarity, accessibility and availability of appropriate documentation and licence indicating the type of reuse permitted (OpenAIRE, 2021). For easy discovery and reuse, good metadata should indicate where, when, what and how the data was collected; be clear for easy interpretation; be well organized; disclose instruments used in collecting data and calibrations used; define the table/row column headings, units of measurement and codes used; explain how to locate and access the data; provide information on what to check if the entire data set was imported; include contact details for clarification; and report strengths, weaknesses and limitations (Michener et al., 1997; Strasser et al., 2012; Zimmerman, 2003, 2007). Information on survey protocols, instruments, experimental processes and methodology is also vital. This too requires “clear and accessible data usage license” (European Commission, 2018, p. 20) to enable third parties to understand the terms and allowable types of reuses permitted. Ultimately, this is facilitated if data is housed through suitable repositories.
The flip side of the FAIR system is the “CARE” principles of scientific-data stewardship to respect and support the interest of farmers and Indigenous Peoples who generate the data. CARE means that the systems should deliver collective benefits, recognize the creator’s authority to control use, be responsible for reporting how data is used, and be guided by the ethical norms of the users and creators (Research Data Alliance International Indigenous Data Sovereignty Interest Group, 2019).
The recent deluge of artificial intelligence (AI) applications may make much of this discussion moot. As AI algorithms search out and train on available data, they begin to lock in on lessons learned and require fewer inputs to begin to operate. Most of the training data has not been formally acquired, which makes negotiations much harder. It is unclear whether this has happened in agriculture, but it is a real risk.
Canada is a major generator of agricultural data but has yet to fully commit to a data-governance system that supports innovation. Data-certification plans such as those operated by the American Farm Board are second-best approaches. While these plans are creating a model for data ownership, without supporting legal and regulatory authority, these are only best-efforts agreements. While laws and regulations cannot define all aspects of governance, clarifying ownership will be necessary at some point. In the absence of legislative direction, markets will either sort this to fit their own interests or courts will be pressed into deciding the matter. Neither approach is efficient. Other markets, like the EU, are already partway along the path to addressing this in legislation. While getting ownership clarified may be necessary, it will not be sufficient to realize the benefits of this technology.
Interoperability needs to be built into the governance system. FAIR and CARE principles provide guidelines, but few governments have made commitments to turn these principles into action. The basic elements are well developed, but they need resources and commitment. There is an opportunity to test this in Genome Canada’s Climate-Smart Agriculture and Food Systems initiative, which is commissioning a data co-ordination and collaboration hub to develop and implement a plan for managing the data generated by nine funded research projects. Depending how this works, it could be adapted to other government-funded research and disseminated as a model to our research and market partners as a platform for better data governance.
Canadian governments have three basic strategic options for dealing with the digital future in agriculture: passive adoption, a proactive muddled approach or ambitious action. As with many problems, we will likely need to trade off feasibility and impact.
The quintessential Canadian strategy for much of what is truly innovative is to just go along with the practices developed around the world. Being a passive adopter and letting others develop and test new applications is a low-risk, low-return option, but it is eminently feasible. The slow rollout of internet connectivity in rural Canada may force this option. In some ways, this makes sense because so much of the investment and effort won’t end up generating returns. It is often a good commercial strategy to let others invest their money and work out the kinks before buying into and using a new technology. But what makes sense at the firm level is not necessarily optimal at the sector- or economy-wide level. Deferring to others will ensure Canada is always playing catch-up, often paying more for technologies that offer a smaller return. There is a sound argument to be made that this option would squander Canada’s comparative advantage and impair global food security.
A more aggressive, albeit muddled, strategy would be to proactively accelerate rural connectivity while at the same time supporting growth in the domestic agricultural-technology provider world by trying to scale our small- and medium-sized startup and growth firms (SMEs). This is the foundation for much of Canada’s innovation policy right now, with “scaling-up” the buzz phrase of the decade. The challenge is that new market entrants and sub-optimally scaled ventures are highly unlikely to raise enough capital to challenge the locked-in data behemoths that dominate the digital agricultural sector. This may simply dissipate our limited development resources on ventures that will never be able to set the global standard for our market segments.
The most ambitious option offers the potential for higher returns but puts more capital at risk. This will require governments — and the federal government in particular — to engage with technology leaders to become a foundational partner in their efforts. This is a high-risk strategy. While Canada is important enough to global agriculture to be worth working with, when push comes to shove, the global ATPs will do what is in their interest. Nevertheless, an ambitious action approach would allow Canada to strategically use its resources to build its interests into their programs, thereby helping to spread the risk relative to working with SMEs. This should generate a higher return for Canada’s agri-food sector because partnerships with global innovation leaders should enable it to push its own interests and, more importantly, to adapt and adopt technologies as soon as they are ready.
The highest return from the digital agricultural play will be the value added on farms and in the domestic supply chain and the related reductions in the environmental footprint of the sector. While it would be nice to get a share of the innovator’s return, this is inevitably going to be a relatively small share of the overall value generated by digitizing agriculture. Moving from a laissez-faire to an ambitious approach is possible but will require policy action on most if not all the policy challenges identified in this paper.
Industry readiness for technology adoption may be a transitory problem, but if left too long, it could dramatically influence how technologies develop and get used in Canadian farming. There are three actionable items that should be addressed in the near term. First, the farm industry is on the cusp of a generational change (the average age of farm operators rose to 56 in 2021), which creates an opportunity to at least partly address the lack of skilled employees. While the Canadian colleges of agriculture and various polytechnics across Canada are turning out highly skilled personnel, few of them are returning to the farm. More can and should be done to improve the skill base in the sector. The Royal Bank of Canada (RBC, 2023) has called for an agricultural skills strategy. In addition to education, there is a need for more just-in-time tailored training by educators and the leading firms producing technology. To be fully effective, incremental training should be certifiable and transferable, which might require selected financial incentives, perhaps similar to some of the other programs offered by Employment and Social Development Canada (such as the Sectoral Workforce Solutions Program).
Second, cyberthreats are real. There was an effort to address security in the food system following 9/11. U.S. Homeland Security Presidential Directives 7 and 9 defined agriculture as critical infrastructure “vulnerable” to terrorist targeting and established a “national policy to defend the agriculture and food systems against terrorist attacks, major disasters, and other emergencies.” Canada, on the other hand, relies on less transparent and formalized policy responses led by the Canadian Food Inspection Agency (CFIA) and targeted more to disease risks at the farm level (CFIA, 2023; Gilpen et al., 2009). There is no explicit discussion of data and system security in either effort. There is an opportunity for Canada to lead the world in this area, but this will require industry, universities, Public Safety Canada and the Canadian Centre for Cyber Security to proactively design a more resilient and secure system. A strategic mix of research, regulation and co-ordination would go a long way to addressing the most vulnerable parts of the system.
Third, governments will need to accelerate efforts to overcome the barriers to widespread technology adoption. This includes expanding rural broadband connectivity to achieve adequate internet speeds in rural and remote agricultural areas. It means considering the creation of an agricultural-data exchange with transparent pricing. Governments can also help counter the market power of large ATPs with more support for homegrown competitors, collaboration with like-minded countries on data regulation, and accelerated adoption of new innovations. The federal government could also draw on lessons learned from Genome Canada’s Climate-Smart Agriculture and Food Systems initiative to develop a data-governance system that supports innovation.
Canada has an opportunity, economic interest and moral imperative to advance any and all technologies that will increase yields, moderate risks and reduce the ecological and environmental footprint of the global food system. Digital applications are the current focus, but success will require a judicious mix of investment, planning and governance that work to link its capacity to amplify and enhance the underlying comparative advantages of the Canadian agri-food system.
AgFunder. (2023). Global agrifoodtech, Investment report 2023. https://research.agfunder.com/agfunder-global-agrifoodtech-investment-report-2023-fb5628814916427c849881af50d068f2.pdf
Agriculture and Agri-Food Canada. (2022a). Overview of the sector. https://agriculture.canada.ca/sites/default/files/documents/2022-07/Sector%20Overview%20-%20Sector%20%282022%29%20EN.pdf
Agriculture and Agri-Food Canada. (2022b). Sector commodity breakdown. https://agriculture.canada.ca/sites/default/files/documents/2022-07/Sector%20Overview%20-%20Commodity%20%282022%29%20EN.pdf
American Farm Bureau Federation. (2014, November 14). Farmers, agriculture technology providers reach agreement on big data privacy and security principles expected to accelerate technology adoption.
American Farm Bureau Federation. (2016). Privacy and security principles for farm data.
Atik, C. (2021). Understanding the role of agricultural data on market power in the emerging digital agriculture sector: A critical analysis of the Bayer/Monsanto decision. In D. Bosco & M. Gal (Eds.), Challenges to assumptions in competition law. Edward Elgar. https://www.elgaronline.com/display/edcoll/9781839109065/9781839109065.00009.xml
Atik, C., & Martens, B. (2020). Competition problems and governance of non-personal agricultural machine data: Comparing voluntary initiatives in the US and EU. JRC Digital Economy Working Paper 2020-07. European Commission. https://www.jipitec.eu/issues/jipitec-12-3-2021/5336/atik_pdf.pdf
Awada, L., Nagy, C., & Phillips, P. (2021). Contribution of land use practices to GHGs in the Canadian Prairies crop sector. PLoS ONE, 16(12), Article e0260946.
https://doi.org/10.1371/journal.pone.0260946
Awada, L., & Phillips, P. (Forthcoming). Market power in smart farming and the distribution of gains in two-stage crop production system. Journal of the Agricultural and Applied Economics Association.
Awada, L., Phillips, P., & Smyth, S. (2018). The adoption of automated phenotyping by plant breeders. Euphytica, 214(8), Article 148.
Balafoutis, A., Beck, B., Fountas. S., Vangeyte. J., Van der Wal. T., Soto. I., Gómez-Barbero. M., Barnes. A., & Eory. V. (2017). Precision agriculture technologies positively contributing to GHG emissions mitigation, farm productivity and economics. Sustainability, 9(8), Article 1339. https://doi.org/10.3390/su9081339
Bayer Global. (2022, February 16). Bayer highlights advancements of agriculture industry’s most prolific R&D pipeline. [News release]. https://www.bayer.com/media/en-us/bayer-highlights-advancements-of-agriculture-industrys-most-prolific-rd-pipeline/
Birner, R., Daum, T., & Pray, C. (2021). Who drives the digital revolution in agriculture? A review of supply-side trends, players and challenges. Applied Economic Perspectives and Policy, 43(4), 1260-1285. https://doi.org/10.1002/aepp.13145.
Bramley, R. (2009). Lessons from nearly 20 years of precision agriculture research, development, and adoption as a guide to its appropriate application. Crop Pasture Science, 60(3), 197-217. https://doi.org/10.1071/CP08304
Bronson, K. (2018). Smart farming: Including rights holders for responsible agricultural innovation. Technology Innovation Management Review, 8(2), 7-14.
Brous, P., Janssen, M., & Vilminko-Heikkinen, R. (2016, September 5-8). Coordinating decision-making in data management activities: A systematic review of data governance principles. In H. Scholl et al. (Eds). Electronic Government. International Conference, EGOV 2016, Vol . 9820 (115-125). Lecture Notes in Computer Science. Springer. https://doi.org/10.1007/978-3-319-44421-5_9
Bullock, D. W., Wilson, W. W., & Neadeau J. (2021). Gene editing versus genetic modification in the research and development of new crop traits: An economic comparison. American Journal of Agricultural Economics, 103(5), 1700-1719. https://doi.org/10.1111/ajae.12201
Burns, C., & MacDonald, J. M. (2018). America’s diverse family farms: 2018 edition. U.S. Department of Agriculture Economic Research Service. https://www.ers.usda.gov/webdocs/publications/90985/eib-203.pdf?v=5435.6
Busse, M., Schwerdtner, W., Siebert, R., Doernberg, A., Kuntosch, A., König, B., & Bokelmann, W. (2015). Analysis of animal monitoring technologies in Germany from an innovation system perspective. Agricultural Systems, 138, 55-65. https://doi.org/10.1016/j.agsy.2015.05.009
Canadian Federation of Agriculture. (2023). Exclusive Starlink discount available. https://www.cfa-fca.ca/11279-2/
Canadian Food Inspection Agency (CFIA). (2023). National biosecurity standards and biosecurity principles. Government of Canada. https://inspection.canada.ca/animal-health/terrestrial-animals/biosecurity/standards-and-principles/eng/1344707905203/1344707981478
Chen, J., & Clark C. (2023). Canadian agriculture at a glance: Canada’s farms were more profitable in 2020 than in 2015. Statistics Canada. https://www150.statcan.gc.ca/n1/pub/96-325-x/2021001/article/00015-eng.htm
Clearwater, R. L., Martin, T., & Hoppe, T. (Eds.). (2016). Environmental sustainability of Canadian agriculture: Agri-environmental indicator report series – Report #4. Agriculture and Agri-Food Canada. https://publications.gc.ca/collections/collection_2016/aac-aafc/A22-201-2016-eng.pdf
Climate FieldView. (2022). Friends with FieldView. https://climate.com/partners/
Dawkins, M. S., Roberts, S. J., Cain, R. J., Nickson, T., & Donnelly, C. A. (2017). Early warning of footpad dermatitis and hockburn in broiler chicken flocks using optical flow, bodyweight and water consumption. VetRecord, 180(20), 499. https://doi.org/10.1136/vr.104066
Dumontier, M., & Wesley, K. (2018). Advancing discovery science with FAIR data stewardship: Findable, Accessible, Interoperable, Reusable. The Serials Librarian, 74(1-4), 39-48. https://doi.org/10.1080/0361526X.2018.1443651
European Commission. (2018). Turning FAIR into reality: Final report and action plan from the European Commission Expert Group on FAIR. https://op.europa.eu/en/publication-detail/-/publication/7769a148-f1f6-11e8-9982-01aa75ed71a1/language-en/format-PDF/source-80611283
Friedman, G. (2014). Workers without employers: Shadow corporations and the rise of the gig economy. Review of Keynesian Economics (2), 171-188.
Gawer, A., & Cusumano, M. A. (2014). Industry platforms and ecosystem innovation. Journal of Product Innovation Management, 31(3), 417-433. https://doi.org/10.1111/jpim.12105
Gilpen, J. L. Jr., Carabin, H., Regens, J. L., & Burden, R. W. Jr. (2009). Agriculture emergencies: A primer for first responders. Biosecurity and Bioterrorism: Biodefense Strategy, Practice and Science, 7(2),187-198. https://doi.org/10.1089/bsp.2008.0037
Group of Seven (G7). (2017). Annex 4: G7 Expert Group on Open Science, executive summary. https://www.g8.utoronto.ca/science/2017-G7-Annex4-Open-Science.pdf
Hall, L. (2017). GPS correction technology lets tractors drive themselves. Tech Briefs. https://www.techbriefs.com/component/content/article/tb/spinoff/environmental-and-agricultural-resources/32572
IEEE. (1990). Standard glossary of software engineering terminology. IEEE Std 610.12-1990, 1-84. https://doi.org/10.1109/IEEESTD.1990.101064
Internet of Food and Farm. (2020). The internet of arable farming. https://www.iof2020.eu/use-case-catalogue/arable
Jobe, G. (2019). Governing value chain disruption in agriculture and agri-food production: A behavioural approach to assessing markets for agricultural data, [Unpublished MPP thesis, University of Saskatchewan]. https://harvest.usask.ca/handle/10388/12440
Kenney, M., & Zysman, J. (2016). The rise of the platform economy. Issues in Science and Technology, 32(3). https://issues.org/rise-platform-economy-big-data-work/
Khatri, V., & Brown, C. V. (2010). Designing data governance. Communications of the ACM, 53(1), 148-152. https://cacm.acm.org/magazines/2010/1/55771-designing-data-governance/abstract
Koutroumpis, P., Leiponen, A., & Thomas, L. D. W. (2020). Markets for data. Industrial and Corporate Change, 29(3), 645-660. https://doi.org/10.1093/icc/dtaa002
Michener, W. K., Brunt, J. W., Helly, J. J., Kirchner, T. B., & Stafford, S. G. (1997). Nongeospatial metadata for the ecological sciences. Ecological Applications 7(1), 330-342. https://doi.org/10.1890/1051-0761(1997)007[0330:NMFTES]2.0.CO;2
Office of the Auditor General. (2023). Connectivity in rural and remote areas. https://www.oag-bvg.gc.ca/internet/English/parl_oag_202303_02_e_44205.html
OpenAIRE. (2021). FAIR — In depth. https://www.openaire.eu/how-to-make-your-data-fair
Organization for Economic Co-operation and Development (OECD). (2020). Issues around data governance in the digital transformation of agriculture – the farmers’ perspective. Working Party on Agricultural Policies and Markets. https://www.oecd-ilibrary.org/agriculture-and-food/issues-around-data-governance-in-the-digital-transformation-of-agriculture_53ecf2ab-en
Organization for Economic Co-operation and Development (OECD). (2021). Data portability, interoperability and digital platform competition. OECD Competition Committee Discussion Paper. https://oe.cd/dpic.
Otto, B. (2011). Data governance. Business and Information Systems Engineering, 3(4), 241-244. https://doi.org/10.1007/s12599-011-0162-8
Pavitt, K. (1998). Technologies, products and organization in the innovating firm: What Adam Smith tells us and Joseph Schumpeter doesn’t. Industrial and Corporate Change, 7(3), 433-452.
Pierce, E., Dismute, W. S., & Yonke, C. L. (2008). The state of information and data governance — understanding how organizations govern their information and data assets. IAIDQ and UALR-IQ.
Pierpaoli, E., Carli, G., Pignatti, E., & Canavari, M. (2013). Drivers of precision agriculture technologies adoption: A literature review. Procedia Technology. 8, 61-69. https://doi.org/10.1016/j.protcy.2013.11.010
Poppe, K., Wolfert, J., Verdouw, C., & Renwick, A. (2015). A European perspective on the economics of big data. Farm Policy Journal ,12(1), 11-19. https://www.researchgate.net/publication/278300518_A_European_Perspective_on_the_Economics_of_Big_Data
Research Data Alliance International Indigenous Data Sovereignty Interest Group. (2019). CARE principles for Indigenous data governance. Global Indigenous Data Alliance. https://www.gida-global.org/care
Rogers, E. (2003). Diffusion of innovations. The Free Press.
Royal Bank of Canada Thought Leadership (RBC). (2023). Farmer 4.0: How the coming skills revolution can transform agriculture. https://thoughtleadership.rbc.com/farmer-4-0-how-the-coming-skills-revolution-can-transform-agriculture/
Sanderson, J., Wiseman, L., & Poncini, S. (2018). What’s behind the ag-data logo? An examination of voluntary agricultural-data codes of practice. International Journal of Rural Law and Policy, 1. https://doi.org/10.5130/ijrlp.1.2018.6043
Shapiro, C., & Varian, H. (1998). Information rules: A strategic guide to the network economy. Harvard Business Review Press.
Shen, S., Basist, A., & Howard, A. (2010). Structure of a digital agriculture system and agricultural risks due to climate changes. Agriculture and Agricultural Science Procedia, 1, 42-51. https://doi.org/10.1016/j.aaspro.2010.09.006
Smyth, S. J. (2022). Contributions of genome editing technologies towards improved nutrition, environmental sustainability and poverty reduction. Frontiers in Genome Editing, 4, Article 863193. https://doi.org/10.3389/fgeed.2022.863193
Smyth, S. J., McDonald, J., & Falck-Zepeda, J. (2014). Investment, regulation, and uncertainty: Managing new plant breeding techniques. GM Crops & Food, 5(1), 44-57. https://doi.org/10.4161/gmcr.27465
Smyth, S., & Phillips, P. W. B. (2003). Product differentiation alternatives: Identity preservation, segregation and traceability. AgBioForum, 5(2), 30-42. https://hdl.handle.net/10355/306
Soto Embodas, I., Barnes, A., Balafoutis, A., Beck, B., Sanchez Fernandez, B., Vangeyte, J., Fountas, S., Van der Wal, T., Eory, V., & Gómez-Barbero, M. (2019). The contribution of precision agriculture technologies to farm productivity and the mitigation of greenhouse gas emissions in the EU. Publications Office of the European Union. https://op.europa.eu/en/publication-detail/-/publication/8309c598-3b0d-11e9-8d04-01aa75ed71a1
Statistics Canada. (2021). Survey of innovation and business strategy. https://www150.statcan.gc.ca/n1/pub/71-607-x/71-607-x2021020-eng.htm
Strasser, C., Cook, R., Michener, W., & Budden, A. (2012). Primer on data management: What you always wanted to know. University of California, Office of the President, California Digital Library. https://escholarship.org/uc/item/7tf5q7n3
Sundmaeker, H., Verdouw, C. N., Wolfert, J., & Pérez-Freire, L. (2016). Internet of food and farm 2020. In O. Vermesan, & P. Friess (Eds.), Digitising the industry — Internet of Things connecting the physical, digital and virtual worlds (pp. 129-150). River Publishers. https://doi.org/10.13052/rp-9788793379824
Terry, S. A., Basarab, J., Luo Guan, L, & McAllister T. (2020). Strategies to improve the efficiency of beef cattle production. Canadian Journal of Animal Science, 101(1), 1-19. https://doi.org/10.1139/cjas-2020-0022
The Peak. (2023, March 27). Starlink’s top competitor takes off. https://readthepeak.com/stories/03-23-starlink-s-top-competitor-takes-off
Ugochukwu, A. & Phillips, P. (2018). Technological adoption by agricultural producers: A review of the literature. In N. Kalaitzandonakes, E. I. Carayannis, E. Grigoroudis, & S. Rozakis (Eds.), From agriscience to agribusiness: Theories, policies and practices in technology transfer and commercialization (pp. 361-377). https://link.springer.com/book/10.1007/978-3-319-67958-7
Ugochukwu, A., & Phillips, P. W. B. (2022). Data sharing in plant phenotyping research: Perceptions, practices, enablers, barriers and implications for science policy on data management. The Plant Phenome Journal, 5(1), Article e20056. https://doi.org/10.1002/ppj2.20056
Van Es, H., & Woodard, J. (2017). Innovation in agriculture and food systems in the digital age. In S. Dutta, B. Lanvin, & S. Wunsch-Vincent (Eds.), Global innovation index 2017: Innovation feeding the world, 10th edition (pp. 97-104). Cornell University, INSEAD/World Intellectual Property Organization.
Verdonk, T. (2019). Planting the seeds of market power: Digital agriculture, farmers’ autonomy, and the role of competition policy. In L. Reins (Ed.), Regulating new technologies in uncertain times (pp. 105-132). Springer.
Weber, K., Otto, B., & Österle, H. (2009). One size does not fit all — A contingency approach to data governance. Journal of Data and Information Quality, 1(1), 1-27. https://doi.acm.org/10.1145/1515693.1515696
Whitt, C. (2022). Farm structure and contracting. U.S. Department of Agriculture Economic Research Service. https://www.ers.usda.gov/topics/farm-economy/farm-structure-and-organization/farm-structure-and-contracting/.
Wilkinson, M. D., Dumontier, M., Aalbersberg, IJ. A., Appleton, G., Axton, M., Baak, A., Bloomberg, N., Boiten, J.-W., da Silva Santos, L. B., Bourne, E. P., Bouwman, J., Brookes, A. J., Clark, T., Crosas, M., Dillo, I., Dumon, O., Edmunds, S., Evelo, C. T., Finkers, R., Gonzalez-Beltran, A., Gray, A. J. G., Groth, P., Goble, C., Grethe, J. S. … Mons, B. (2016). The FAIR Guiding Principles for scientific data management and stewardship. Nature, 3, Article 160018. https://doi.org/10.1038/sdata.2016.18
Wiseman, L., Sanderson, J., Zhang, A., & Jakku, E. (2019). Farmers and their data: An examination of farmers’ reluctance to share their data through the lens of the laws impacting smart farming. NJAS — Wageningen Journal of Life Sciences, 90-91(1), Article 100301. https://doi.org/10.1016/j.njas.2019.04.007
Wixted, B. (2009). Innovation system frontiers: Cluster networks and global value. Springer Science & Business Media.
Wolfert, S., Ge, L., Verdouw, C., & Bogaardt, M. J. (2017). Big data in smart farming — A review. Agricultural Systems, 153, 69-80. https://doi.org/10.1016/j.agsy.2017.01.023
Yeates, J. W. (2017). How good? Ethical criteria for a ‘Good Life’ for farm animals. Journal of Agricultural and Environmental Ethics, 30(1), 23-35. https://doi.org/10.1007/s10806-017-9650-2
Zimmerman, A. S. (2003). Data sharing and secondary use of scientific data: Experiences of ecologists [PhD dissertation, The University of Michigan]
Zimmerman, A. S. (2007). Not by metadata alone: The use of diverse forms of knowledge to locate data for reuse. International Journal on Digital Libraries, 7, 5-16. https://doi.org/10.1007/s00799-007-0015-8
Zuboff, S. (1985). Automate/lnformate: The two faces of intelligent technology. Organizational Dynamics, 14(2), 5-18. https://doi.org/10.1016/0090-2616(85)90033-6
This paper is the first in a three-part IRPP research publication and lecture series, Imagining a Better Digital Future for Canada, sponsored by TELUS. It was developed under the direction of the vice president of research, Rachel Samson, supported by senior writer and editor Rosanna Tamburri. The manuscript was copy edited by Shannon Sampert, proofreading was by Zofia Laubitz, editorial co-ordination was by Étienne Tremblay, production was by Chantal Létourneau and art direction was by Anne Tremblay.
Peter W. B. Phillips is a distinguished professor emeritus of public policy and founding director of the Centre for the Study of Science and Innovation Policy at the University of Saskatchewan’s Johnson Shoyama Graduate School of Public Policy.
To cite this document:
Phillips, P. W. B. (2023). Can Canada Help Feed the World While Reducing Emissions? Assessing Challenges and Barriers to Digital Opportunities in Agriculture. IRPP Insight No. 50. Montreal: Institute for Research on Public Policy.
This brief draws heavily on work co-produced with L. Awada, J. Relf Eckstein, S. J. Smyth and A. I. Ugochukwu.
The opinions expressed in this paper are those of the author and do not necessarily reflect the views of the IRPP, its Board of Directors, or sponsors. Research independence is one of the IRPP’s core values, and the IRPP maintains editorial control over all publications.
IRPP Insight is a refereed series that is published irregularly throughout the year. It provides commentary on timely topics in public policy by experts in the field. Each publication is subject to rigorous internal and external peer review for academic soundness and policy relevance.
If you have questions about our publications, please contact irpp@nullirpp.org. If you would like to subscribe to our newsletter, IRPP News, please go to our website, at irpp.org.
Cover photo: Shutterstock.com
ISSN 2291-7748 (Online)
Montreal – Food security and affordability are a concern across Canada, and around the world. New research from the IRPP outlines how the adoption of a digital strategy could help Canada’s agriculture sector reduce hunger, adapt to climate change, optimize land and water use, and promote sustainable economic growth across the country.
New technologies are emerging such as precision agriculture, variable-rate technology, digital-farm management and digital animal-health management, which hold the potential to benefit farmers and consumers. But several hurdles stand in the way.
In a paper published by the IRPP, University of Saskatchewan professor emeritus Peter Phillips calls on Canadian governments to adopt an ambitious high-risk, high-reward strategy that will overcome these barriers and optimize the use of these promising technologies. The strategy requires bold action and collaboration from governments, the private sector and academic institutions. Phillips recommends that policymakers:
“Governments should take advantage of the significant opportunities for economic growth for technology developers, telecommunication companies, farmers and other companies along the supply chain from farm to table,” says Phillips.
“Canada has the chance to succeed with a well thought out plan and the right mix of investment, planning, collaboration and governance. Canada can do well by doing good, increasing our economic returns from our world-class agri-food system, and contributing to global food security and climate change goals. There is no time to waste,” Phillips concludes.
This publication is the first in a three-part series, Imagining a Better Digital Future for Canada. The series was made possible with the support of TELUS.
Can Canada Help Feed the World While Reducing Emissions? Assessing Challenges and Barriers to Digital Opportunities in Agriculture can be downloaded from the IRPP’s website (irpp.org).
Media contact: Cléa Desjardins – 514-245-2139 – cdesjardins@nullirpp.org